What are Rare Earths?

The Japanese call them “the seeds of technology.” The US Department of Energy calls them “technology metals.” They make possible the high tech world we live in today – everything from the miniaturization of electronics, to the enabling of green energy and medical technologies, to supporting a myriad of essential telecommunications and defense systems. They are the elements that have become irreplaceable to our world of technology owing to their unique magnetic, phosphorescent, and catalytic properties.
Which Elements are They?
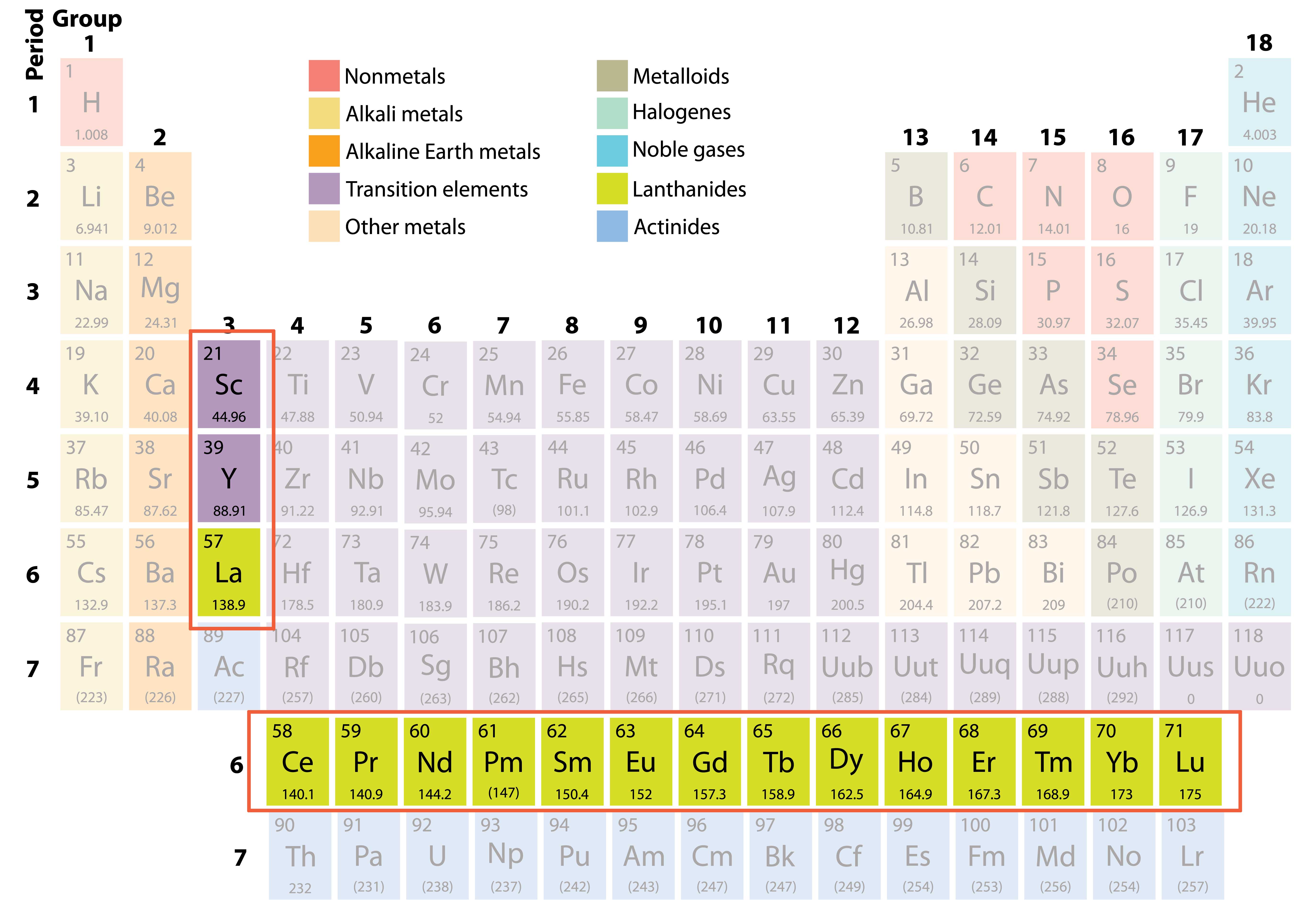
Rare earth elements are a set of seventeen chemical elements in the periodic table, specifically the fifteen lanthanides plus scandium and yttrium. Scandium and yttrium are considered rare earth elements since they tend to occur in the same ore deposits as the lanthanides and exhibit similar chemical properties. While named rare earths, they are in fact not that rare and are relatively abundant in the Earth’s crust. What is unusual is to find them in quantities significant enough to support economic mineral development.
With rare earths, a little goes a long way. The amount of rare earths used in high tech equipment is nominal but almost always critical to the unit’s performance. For example, an iPhone uses eight rare earths – for everything from its colored screen, to its speakers, to the miniaturization of the phone’s circuitry. While the amount of rare earths in each phone is very small, the quantity of phones sold each year is impressive. According to Apple, in 2012 over 125 million iPhones were sold worldwide, up from 72 million in 2011.
The rare earth elements (REEs) are comprised of the lanthanide elements plus scandium and yttrium, which have similar physical properties and are often found in the same ores and deposits. Specifically, REEs include the light REEs (LREEs) such as lanthanum, cerium, praseodymium, neodymium, samarium, europium, and the heavy REEs (HREEs) gadolinium, terbium, dysprosium, holmium, erbium, thulium, ytterbium, lutetium, scandium and yttrium.
While most of these elements are not actually rare in terms of general amount of these elements in the earth’s crust, they are rarely found in sufficient abundance in a single location for their mining to be economically viable. REEs have many important applications in modern technology for which there is no equal substitute, but an increasing demand for these elements is straining supply.
Properties and Application
The physical similarities in atomic radius and charge between REEs led to their separation as distinguishable elements. However, REEs have not found a common technological use until recent advances made separation economically feasible. As stated earlier, these REEs are also low in abundance in rock deposits, further increasing difficulty in their separation. Combined with their low abundance in many rock deposits, this ensured that REEs have not found a common use in technologies until recent advances made separation economically feasible. Their similarity means that some make good substitutes for one another but for the group overall the applications vary greatly.
LREEs such as Ce show a strong affinity for elements like phosphorus, making them suitable as water purifiers, while La acts as a hydrogen absorber in rechargeable batteries. In addition, both of these elements act as stabilizers in catalytic compounds, such as in the process of transforming oil to gasoline. Cerium is also present in catalytic converters in automobiles, sometimes up to 30 (wt)%. Lanthanum is an important element in hybrid car batteries; each Prius on the road contains about 4.5 kilograms (Koerth-Baker, 2012).
REEs such as Eu, Y, and Tb are used to produce more energy-efficient phosphors than halophosphors, which are composed of Sb and Mn, in the displays for computers and phones, as well as in compact fluorescent lamps. Erbium is used in the making of fiber optic cables and laser repeaters. (Tiesman, 2010)
REEs such as Nd and Dy can handle a greater saturation magnetization than more common elements like iron, which allows for fabrication of a stronger and smaller magnet. Combined with other elements, these magnets (Nd-Fe-B magnets) are among the strongest magnets in the industry, able to withstand temperatures as high as 230 C and operate at a smaller size than gearboxes in wind turbines and hybrid cars. Dysprosium is also critical to high performance in electric motors from surgical robots to wind turbines (“The supply/demand dynamics,” 2010).
Overall, REEs have applications in technologies ranging from iPods to general household appliances to military defense systems. They are integrated into every part of modern life, from light bulbs to cars. Although used in small quantities, without them many technologies would not function as well. Additionally, their uses in environmentally-beneficial technology puts them at a high priority for ameliorating environmental concerns.
A primary worry is that middle class families (growth of which is correlated with development of a country) are growing reliant on these products. Developing countries would not become fully developed if supply of these elements dropped, as they would not have access to technologies like better refrigerators, computers or clean energies such as wind turbines. Even if the products are not necessary, the demand for production of REEs can yet stimulate a developing economy as not only a raw materials supplier, but also as an end-product producer.
Though only used in trace amounts in most of their applications, the demand for REEs has increased exponentially, with the current yearly production roughly equal to the total production before 1950 (Hatch, 2012). The supply must be increased if we are to continue day-to-day usage of these elements.
Geology and Mining
Rare earth elements occur in many minerals but typically in concentrations too low to be refined in an economical manner; the concentration of REEs in the Earth’s crust is estimated to be between 150 to 220 parts per million, which is higher than the concentration of other metals mined for industrial use, such as Cu or Zn. The concentration of cerium, the most abundant rare earth, is approximately the same as that of copper although the other REEs are much rarer. However, REEs are not usually concentrated in ore deposits in amounts that can be easily or economically mined (Long, 2010).
Economically exploitable REE concentrations are generally found in uncommon types of igneous rocks such as carbonatites and alkaline rocks. They can also be found (as parts of mineral compounds) in placer deposits, residual deposits due to weathering, pegmatites, iron-oxides copper-gold deposits, and even in marine phosphates (Long, 2012). Assaying, or quantitative and qualitative assessment of a mineral, is a common method for determining the mineral concentration, but this is costly. Coupled with the scattered nature of deposits, exploration companies are still searching for suitable locations to mine (see Locations of Deposits page). An additional difficulty is the price volatility of the REE market, especially in the recent years.
Something that must also be taken into account when considering REEs is the strategic value of having mineable quantities of these elements. For instance, China’s deposits provided it with leverage of global markets of these elements. Before 2010, China controlled 95% of the production market of REEs (Chakhmouradian, 2012). Now that new deposits are being discovered around the globe, and new refineries are opening up (for HREEs in particular, of which China previously provided 100%), this monopoly may cease.
Refining
Refining involves separation of an element from its ore through the removal of impurities. So as to decrease the cost of transportation of the huge quantity of ore needed to refine, refining is often done at the mine itself. Refinement occurs through physical separation of the REEs by various chemical techniques, sometimes involving thousands of steps. Unfortunately, all REEs and their respective ores are different and require different chemical techniques for refining (depending on melting point and vapor pressure, as well as other physical properties of the element).
Typical techniques for refinement include milling, where ore is ground down to fine particles and then separated in a variety of ways, followed by cracking, where the it undergo a series of chemical treatments. Due to the large number of steps the REEs must go through to be purified requiring many different chemicals and reagents for these processes, there is a huge amount of toxic and radioactive waste generated from byproducts, which must be handled either through recycling, or by pumping it to a holding tank. The waste presents various health and environmental issues that must be dealt with at the mining site. In addition, the amount of processing that goes into purifying REEs incurs a huge cost, and raises the price of the element significantly
Where do They Come From?
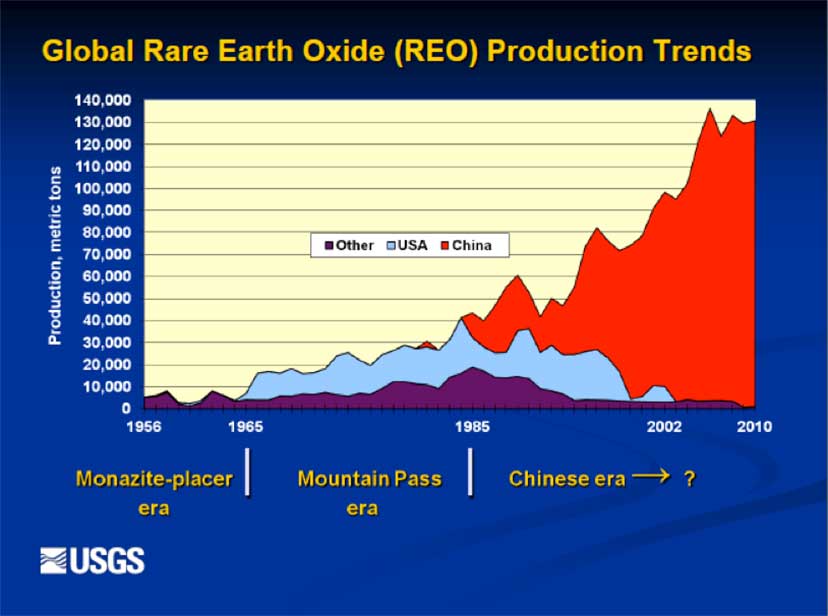
Since the 1980s, China has produced roughly 90% of the world’s supply of REEs (Chakhmouradian, 2012). It currently produces 97% of the world REE supply, giving China immense bargaining power. They have recently slowed exportation to Europe and the Americas, and they have stopped exporting to Japan (Tiesman, 2010). With these exportation decreases, world prices skyrocketed up to 1500% over the course of just a few months (Hatch, 2012). Countries that manufacture REE-containing products were therefore dependent on China’s willingness to continue to export to them. However, recent geological surveys from Britain and the U.S. indicate that reserves of REEs may be found in many diverse locations around the world, and some REE-dependent manufacturers are financially backing further exploration of potential reserves outside of China (Chakhmouradian, 2012). Demand is still increasing, but supply now exists outside of China (Mt. Weld in Australia and Mountain Pass in California) and more mines should open around the globe as time goes on, particularly in Brazil, Australia and Russia.
China became the world’s dominant producer of rare earths in the 1990s. Because China sold rare earths at very low prices, mines like Molycorp’s Mountain Pass in California and others throughout the world were unable to compete. By 2000, China accounted for more than 95% of world rare earth production.
China is also the dominant consumer of rare earths, which they use mainly in the manufacture of electronics products for domestic use as well as export. Japan and the United States are the world’s second and third largest consumers of rare earths. In 2010 and 2011, Chinese exports of rare earths fell, driven predominately by increasing domestic consumption. China also announced they may require imports by 2014 of certain rare earths, some that are deemed “critical” by the U.S. Department of Energy1
In a normal market, supply reductions would trigger price increases. At that point, new sources would be developed. The long lead time between discovery and production means there is no quick way to increase supply in the rare earth market. End-users are at a significant disadvantage by only having one major supplier. (Other sources are being developed, including the Bear Lodge Project, but none are currently in full production or can provide a full suite of all the rare earth elements.) These factors have resulted in a great deal of uncertainity in the market place and significant price volatility.
Why the REE are very important?
Rare Earth Elements (REE) are metals having many similar properties. The global demand for rare earth elements has increased significantly in line with their expansion into high-end technology, environment, and economic areas. In this post, we see the importance of Rare Earth Elements and their strategic significance.